Nuclear power accounts for nearly 20% of American electricity generation, which still outpaces renewable power generation by a few points. That output is done at legacy facilities since the United States has not completed a new nuclear power plant in three decades. However that appears likely to change soon, and nuclear power can be a key part of decarbonizing our energy mix and reducing air pollution.
On October 16th, the Department of Energy approved a multi-year cost share award of up $1.355 billion to a new entity, the Carbon Free Power Project, to demonstrate and deploy a 12-module small modular reactor (SMR) power plant. This news follows the first ever SMR design to receive U.S. Nuclear Regulatory Commission Design Approval in August. NuScale Power’s design is forecasted to be built by and start operation in the Carbon Free Power Project plant in 2029.
The 720 megawatt (MWe) fission plant will be located at the Idaho National Laboratory and be composed of NuScale’s 60 MWe nuclear power modules. If the process goes well, Utah Associated Municipal Power Systems (UAMPS), which owns the Carbon Free Power Project, hopes to begin construction of the plant in 2025. Applications for construction and operation are set to be applied for in 2023. UAMPS will be distributing energy from the plant to their participating public power utility customers in Utah, California, Idaho, Nevada, New Mexico, and Wyoming. UAMPS CEO noted that this project will complement and enable additional intermittent renewable energy, wind and solar that are a growing part of the agency’s portfolio.
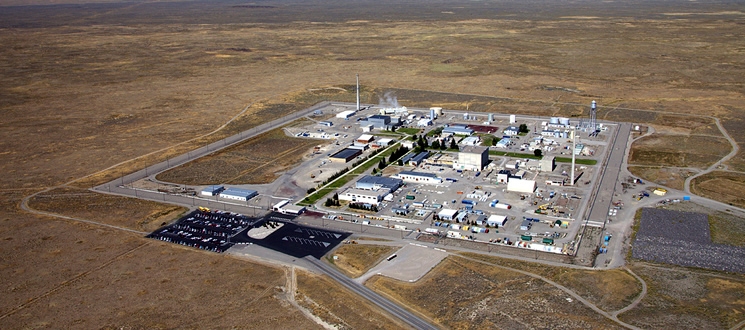
The SMR power plant will be the first of its kind in the United States, whose fleet of fission reactors has begun a slow string of retirements. Nuscale’s small form factor fission reactor technology is a part of a newer family of Generation III/III+ reactors, which have better economics and safety features compared to the Generation II reactors that are stereotypical of the American fleet and public perception.
Supposed benefits of SMRs and their improvement in fission energy may make them compelling options for growing urban communities in search of non-carbon energy sources. If the technology proves to live up to its promises, it could revive America’s nuclear industry and help provide our cities with fossil free power. Local fission and climate advocates at Seattle Friends of Fission (FoF) see great hope in this form factor of advanced nuclear.
Where SMRs depart from conventional nuclear
As defined by the World Nuclear Association, small modular reactors are nuclear fission reactors generally 300 MWe equivalent or less, designed with modular technology using module factory fabrication, pursuing economies of series production and short construction times. These properties and modern reactor design potentially allow SMRs to avoid the older and prototypical reactors’ baggage, namely safety, cost overrun, and construction delay concerns.
Chernobyl, Three Mile Island, and Fukushima meltdowns immediately come to mind when thinking of safety and nuclear. Products of a combination of negligence, human error, and design, these incidents greatly reduced the favorability of nuclear energy at the time. These systems required active cooling systems, which ended up failing in spectacular meltdown and radiation contamination.
Studies of Chernobyl, Three Mile Island, and Fukushima debate the actual scale or lack of long-term health consequences of actual meltdown. Even in the case of Chernobyl (the most catastrophic meltdown in history) drawing causal links from radiation exposure to upticks in solid cancers or change in fertility rates and birth defects has been elusive. Risks have been primarily associated with nuclear workers exposed to high levels of radiation and children exposed to I-131 suffering a higher rate of thyroid cancer.
Radiation exposure is certainly dangerous, but the human body is more resilient to it than we thought. That said, the mental health consequences of meltdowns can be severe. Botched evacuation and relocation measures seem to be the primary cause of public health consequences for Fukushima’s population. Despite scientific push back, the danger of meltdown rates high in public perception.
Modern SMRs promise the erasure of the need for an active cooling system. Potential quality control benefits from factory production paired with third generation nuclear reactor developments can make for an inherently safe reactor. NuScale’s design leverages the smaller scales of SMR to enable a focus on passive or inherent safety that based on simulations cannot meltdown–a claim supported by the Nuclear Regulatory Commission, the strict regulatory body for nuclear energy in the US. The system will naturally circulate water to cool the core without the need for pumps, AC or DC power, human/computer intervention, or external water.
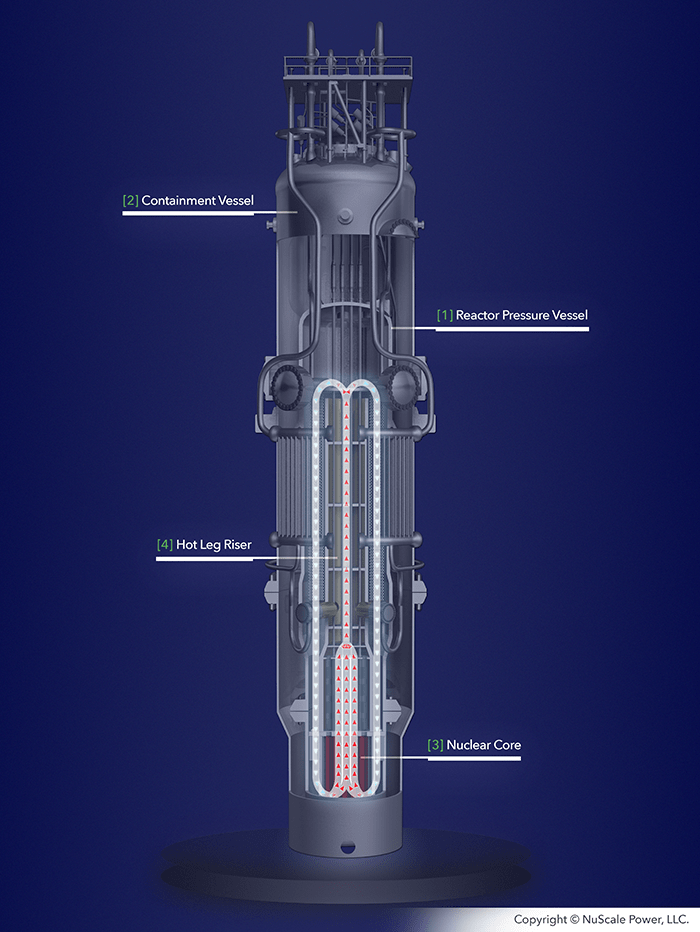
On cost and time overruns, large reactors can attribute that problem to their effectively megaproject status. The typical form factor of fission reactors are rated around 600 to 1200 MWe each, or enough electricity to power around 400,000 to 800,000 American homes. This kind of scale’s long construction timelines and costs threaten to grow with risk factors. Delays often arise from productivity, plant engineering, and design issues, which often cause an average five-year timeline to increase by years–decades if funding comes a problem. Further complications arise from the diseconomies of production, as new reactor construction in the states utilize different designs and is done sporadically.
This delay is currently being seen in Georgia where Vogtle Unit 3 and 4. The greater plant was already home to two older units– have seen a constant series of delays. Originally planned to be operational in 2016 and 2017, these dates have now been pushed to 2021 and 2022. It has also gone billions over budget. These problems were caused by overly optimistic productivity targets and now Covid-19 reducing their workforce. This kind of delay scares utilities that may have to saddle their consumers with driven up utility bills to offset overruns.
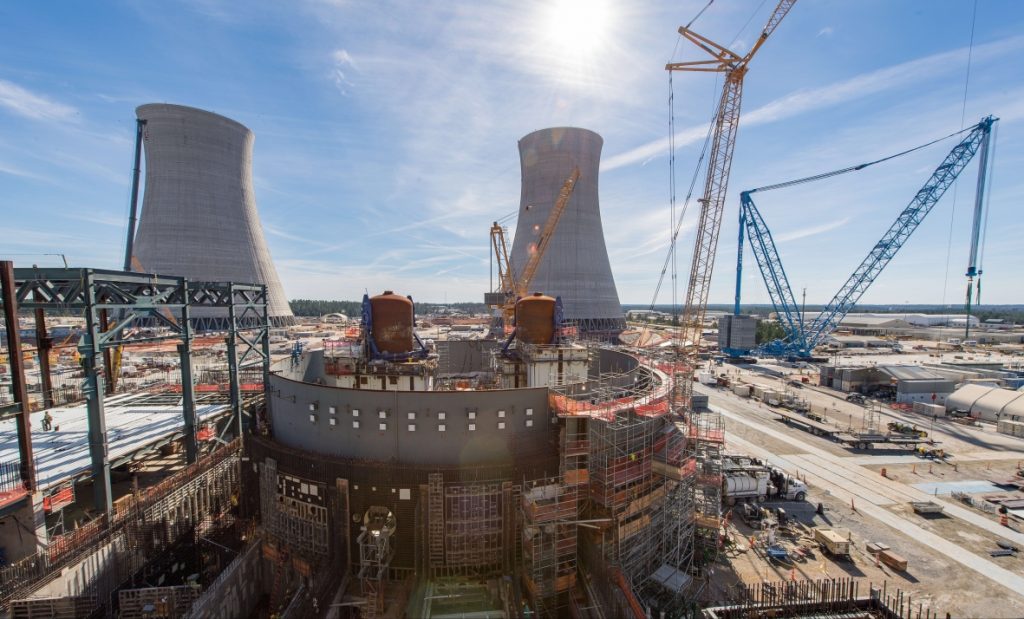
SMRs exchange power output for greater simplicity of design and a smaller footprint that allow for production efficiencies in factories, shorter construction times, and reduced citing costs. NuScale claims that its SMR design can be built in around two years, and believes that it can meet a 2027 timeline for the UAMPS power plant. Note that the aforementioned 2029 start is at the direction of the customer that has to align with its member needs and further derisk the project. Given that this would be the first SMR power plant built in the United States, much uncertainty surrounds the potential risks and the claims of economies of scale applying to the technology.
The general idea is that factory production enabled by the smaller size allows SMRs to share the benefits of prefabricated construction that addresses productivity, engineering, and design issues in large reactors. With regular production, potential economies of series production help eliminate cost and safety issues over time, as a manufacturer becomes better at manufacturing identical reactor designs, is able to order materials in bulk, and avoid environmental conditions of reactor construction on site.
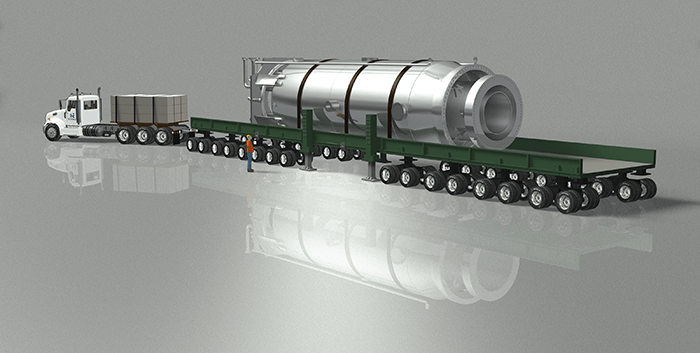
While they may not be as powerful as their larger counterparts, small reactor plants can be flexibly scaled up by adding another SMR to the power plant to match or overtake a traditional power plant. Having more and smaller reactors also increases the flexibility of a power plant. Scaling up or down can be done to match the energy needs of serviced communities. Maintenance of a reactor is less impactful because one small offline reactor is not a major deal compared to a large reactor going offline.
Measuring small nuclear up against other energy sources
When compared to renewables, SMRs clearly fall into a different niche for energy generation. These small reactors are a non-intermittent, energy dense, and mostly safe option. The renewable mix of sources each have their own variations on intermittency, energy density, manufacturing, and safety concerns. Where each type is used will have to depend on the needs of the served community.
Using a term that urbanists have an affinity for, density is the primary advantage of nuclear energy. Density in this case refers to the amount of energy a power source is able to produce in an area. Nuclear fission without a doubt is the most energy dense power source available to us right now. For scale, the 12 module NuScale power plant will be able to power half a million homes and cover less than a tenth of a square mile.
For solar, meeting a third of US energy needs by 2050 will take 11 thousand square kilometers (4250 square miles), or 4 thousand square kilometers (1550 square miles) if all the panels were in Arizona, industry-leading 21% efficient, and with horizontal installation with complete ground coverage as well. Calculating that number for wind is trickier, as there are vast swathes of land between each turbine. Jesse Jenkins, an assistant professor at one of Princeton’s engineering schools, found that 66,000 square kilometers (25,500 square miles) of wind farm would be needed for a third of US energy needs by 2050, but only 700 square kilometers (270 square miles) would be permanently taken up by production. The number for solar land use is also mitigated if coverage is distributed across rooftops for solar, but it does not eliminate the significant land usage of either of the two renewables.
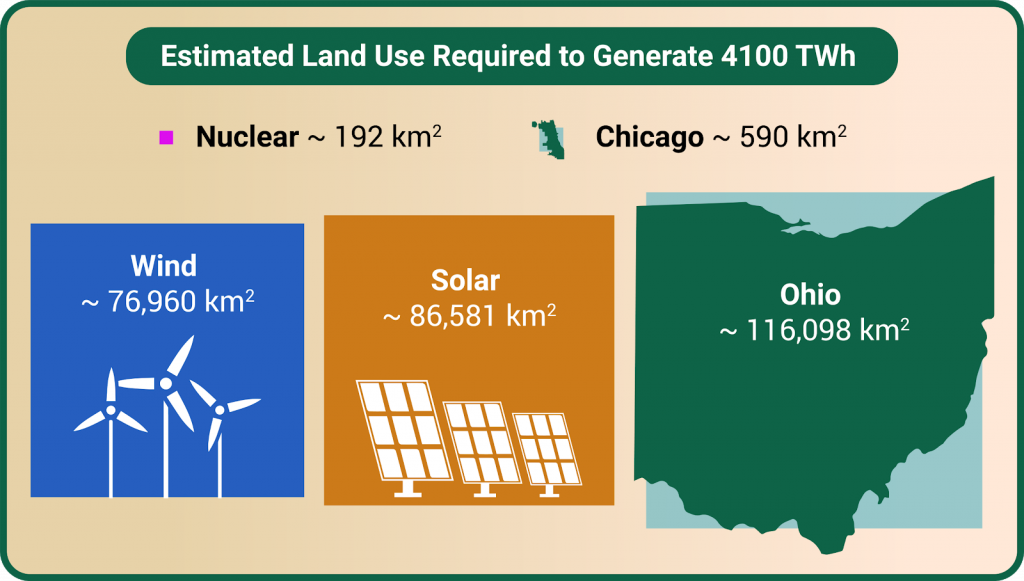
For conventional fission power plants that include buffer zones and cooling ponds, only 440 square kilometers (170 square miles) are needed to meet a third of US energy needs by 2050, 1500 square kilometers (580 square miles) if you include mines and waste storage–factors not included in the renewable numbers. Given how energy dense the technology is, Seattle Friends of Fission‘s Karl Pauls suggested expansion of our state’s Columbia Generating Station in Eastern Washington. The singular station already provides for 10% of Washington’s electricity needs and could likely house more reactors, eliminating the need to get into the politics of citing a new nuclear plant.
A small footprint for nuclear energy also accounts for less waste by volume compared to less dense energy sources. Wind turbines last around 20-25 years, and solar panels last around 25-30 years. With recycling capability for wind turbines and solar panels poor right now, a significant amount of waste will go to the landfill right now. The complexity of solar panels makes them harder to recycle. As they break down, solar panels can leach toxic metals. By 2050, the International Renewable Energy Agency expects the world to have a stock of 78 million tons of panel waste in need of recycling infrastructure.
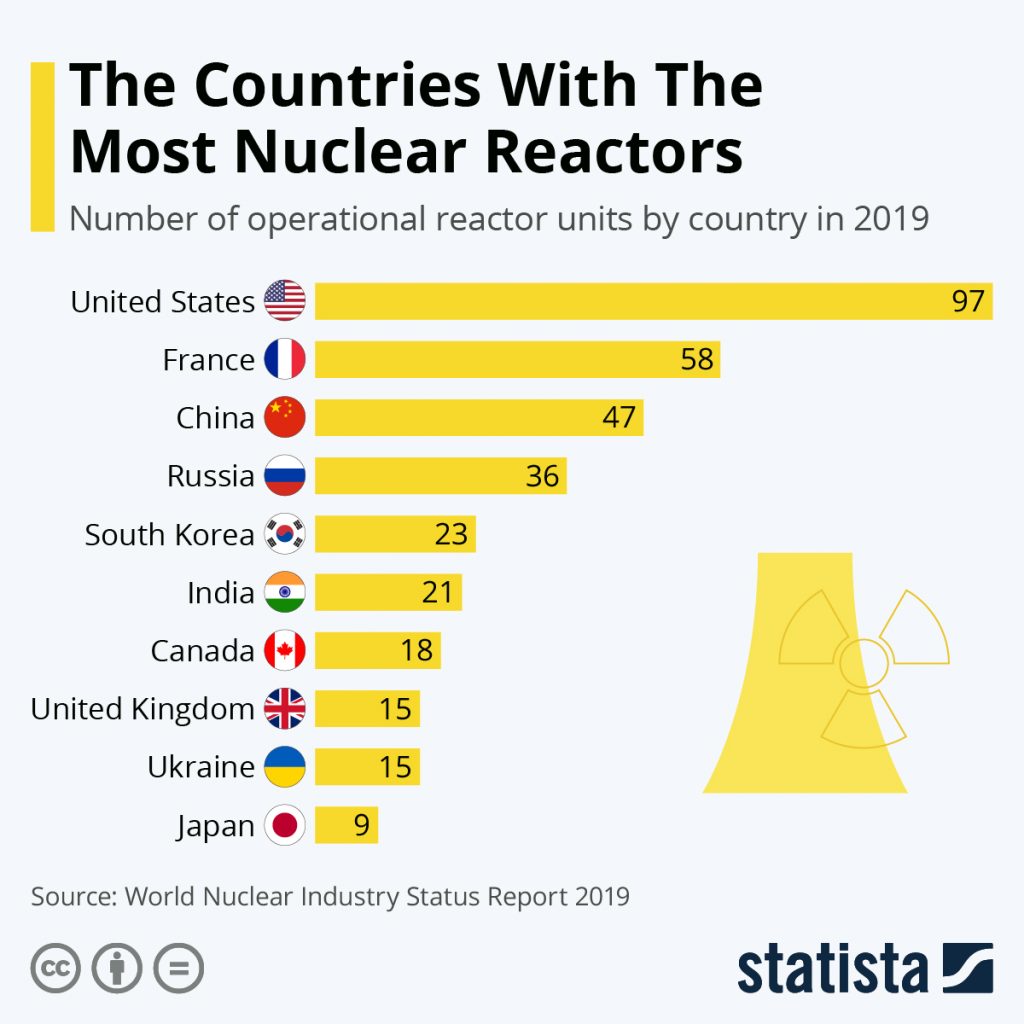
Spent nuclear fuel poses a major issue, but by volume there is much less to be recycled or stored. Currently, nuclear waste is stored on-site. Politics prevented Yucca Mountain from becoming a permanent disposal site. This is probably for the best, as it was a poor location in the first place due to Nevada being the 4th most seismic state, and the multiple fault lines running somewhat close by.
There’s also the WIPP site, the only operating deep geological repository in the world. It’s located in an ideal location in New Mexico’s salt flats that are nearly impermeable–preventing access to the surface or groundwater–and geologically stable. The facility currently holds transuranic waste that includes reprocessing and nuclear weapons waste. It’s capable of safely holding spent fuel, but it’s only licensed to hold transuranic waste right now. Some advocates of a centralized repository for nuclear waste want WIPP expanded to allow spent fuel storage and believe that the facility is capable of holding all of America’s existing and future nuclear waste (skip to 39:00).
Baseload versus a flexible grid
On intermittency and variability, SMRs produce non-intermittent and constant levels of energy. How important this factor is dependent on the energy infrastructure of a system, the nation’s current and unsophisticated infrastructure demands the stability that non-intermittent energy sources like nuclear fission, hydro, and carbon power plants provide. Nuclear could easily substitute fossil fuels with existing infrastructure, which poses a hurdle for high renewable penetration.
This topic of intermittency is often used to pit nuclear energy’s non-intermittent and constant levels of energy, or sometimes called baseload energy, against intermittent and variable renewable energy sources. Some environmentalists want to move away from the idea of baseload energy in favor of a much more flexible energy system that can fully accommodate an entirely renewable portfolio.
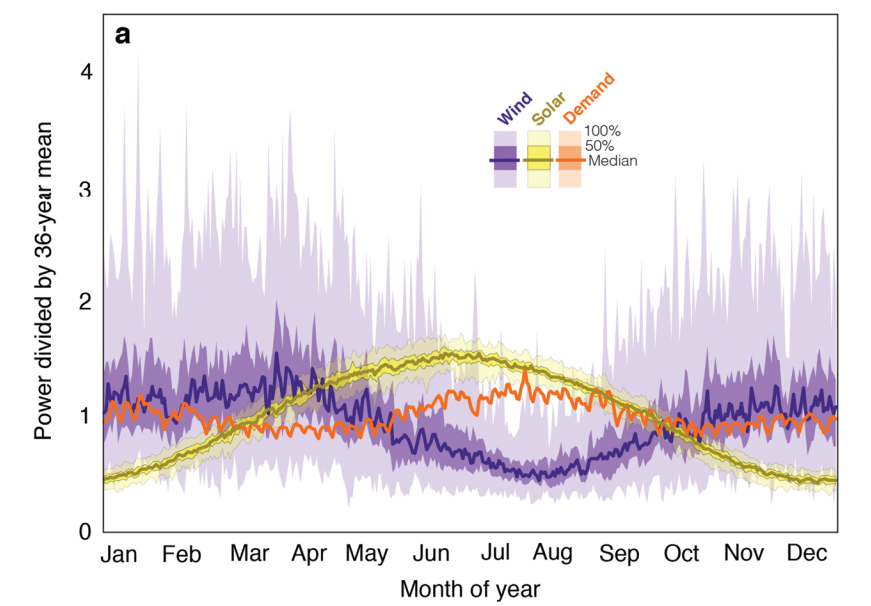
A flexible grid is a more intelligent and comprehensive energy grid system, which entails energy storage systems and smart grid improvements that would be needed to have the flexibility that high capacity wind and solar energy need to function reliably. An in-depth 2018 peer reviewed paper, published in Energy & Environmental Science, generated data on the reliability of 100 percent solar, wind, and combined scenarios with different storage capacities. The authors found that meeting 80% of US energy demand was realistic with a wind and solar mix and reasonable storage capacity. Bridging the last 20% requires drastic storage capacities or overproduction on a massive scale.
Smart grid systems can help with that last 20% by managing electricity supply and demand. In practice, that management could look like deliberate power shut offs of low priority uses like car battery charging. These systems and energy storage are an additional and high cost. That being said they are important improvements that our grids can use to be more efficient. The flexible grid approach certainty has merit, but it requires a transformation of the US energy grid, and probably substantial, sustained, public, and political will.
If we can’t change our grid system, then SMRs are positioned to be the ideal baseload energy provider. Coal and natural gas plants can easily be substituted by small nuclear power plants. Pauls of Seattle FoF also pointed out that costs could be saved in this kind of scenario because all the grid infrastructure could just be reused, as nuclear operates off a similar grid model as fossil plants.
Challenges
Public sentiment poses the greatest challenge to the viability of nuclear power. Gallup polling in 2019 had nuclear power equally favored and opposed by 49% of the American public. On emphasis, Gallup found that roughly a third of surveyed Americans supported more, less, or the same emphasis each. The pollster correlated perceptions on cost and safety with nuclear power’s favorability, which declines whenever an incident occurs or when oil is cheap. These numbers certainty aren’t bleak as one might imagine, but Gallup found that its renewable counterparts to be much more popular with their cleaner image and now rosy economics.
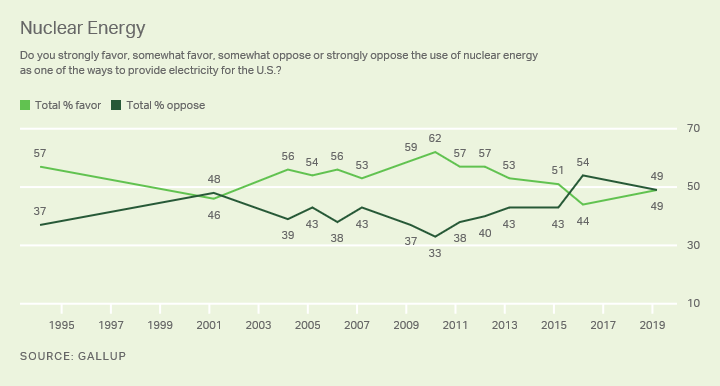
Especially with the cost of solar plummeting to the cheapest form of energy in the states with market competition and government subsidies, SMRs will have to compete in a crowded market. A traditionally large nuclear plant offers competitive prices for electricity, but upfront and major capital costs and risk of overrun costs and delays scare off investors and public utilities that may have to suffer the wrath of their constituency. SMRs lower that barrier of entry, but its economics are still unproven as none have entered the commercial market. If economies of scale and other potential cost efficiencies do prove price competitive with other energy sources, then dispelling misconceptions on safety will also have to occur for wide-spread SMR adoption and revival of the US nuclear industry.
While fission does have inherent risk, decades of technological development on reactor safety shouldn’t be ignored. NuScale’s SMR design incorporates these safety developments with a design that can provide core cooling for an indefinite period of time and cannot meltdown. The U.S. Nuclear Regulatory Commission also keeps the industry in check with standards that make working in the nuclear industry one of the safest, with the least deaths per trillion kilowatt hours attributed to nuclear power among energy sources. Coal is the most deadly energy source, but oil isn’t far behind–and that’s before factoring in climate change.
Where does small nuclear belong
Whether or not SMRs fit into the niches demanded by our urban areas, it will have to depend on the needs of each community to develop energy portfolios. As the Seattle area is now, hydropower is our main source of electricity, with wind power as a secondary renewable generator. Nevertheless, the region still burns coal and natural gas. While Seattle City Light is overwhelmingly non-carbon, Puget Sound Energy’s electricity generation relies on natural gas and coal for a portion of its generation that varies in the tens of percentage points as demand and renewable generation fluctuates.
While it would take around five to eight years for it to come online, new nuclear capacity–SMR or conventional–is a powerful option that can swiftly ease us off fossil generated electricity. Bolder capacity increases could help our carbon reliant neighbors reduce their emissions with interstate electricity exports. It also gives our region the time to start the process of modernizing our grid and continue expansion of renewable generation without having to rely on fossil back ups or even hydropower dams that wreak havoc on our ecosystems.
Furthermore, if you expect the region’s population to swell then new energy demands must be accommodated with reasonable tradeoffs. This is where more nuclear energy may be needed to accommodate that energy demand. Expansion of hydro power is limited and controversial in Washington, and extreme expansion of other renewables would demand major improvements to our energy infrastructure that may not be feasible soon enough.
Small modular nuclear reactors may fit the needs of urban utilities that are in need of a tremendous amount of energy, and have limited ability to reliably expand their other non-carbon energy sources. Where the SMR plant, and its eventual waste is placed then becomes the question. Given how safe the technology potentially is, the reactor could be placed close to the consumers to save on transmission costs and reduce energy losses from transmission, but that could inflame public fears on the energy source. Expansion of existing nuclear power sites or replacement of fossil plants with nuclear could be how we approach nuclear energy expansion.
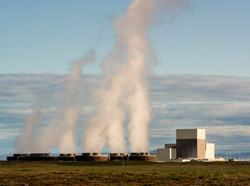
Energy and urbanism is inextricably linked. Our cities are wholly dependent and major consumers of electricity. We will only become more dependent and more electricity-hungry as cities continue to electrify away from carbon emitters. Our dependence on electricity demands the utmost in energy security and small nuclear reactors may be able to meet those demands without many of the downsides of their larger counterparts.
Within the relationship of energy and urbanism also lies equity and public health issues, BIPOC and other vulnerable communities are often close to or have polluting fossil power plants within them. There is also the responsibility to our Indigenous neighbors to restore their waterways and ecosystems, which could be done with replacing dams with nuclear plants, solar arrays, and wind turbines. A cast of renewables supported by SMRs could also allow cities to take steps toward equity and public health goals on top of their energy security need.
Author’s Note: The word fission is often used rather than nuclear at times because I want to specifically name the nuclear process utilized in SMRs. I also want to separate it from and hold out hope of fusion energy, the elusive unicorn cousin of fission that makes the sun shine, and makes many of our renewables possible. I would also like to extend thanks to local advocates at Seattle Friends of Fusion for their aid on the topic.
Shaun Kuo is a junior editor at The Urbanist and a recent graduate from the UW Tacoma Master of Arts in Community Planning. He is a urban planner at the Puget Sound Regional Council and a Seattle native that has lived in Wallingford, Northgate, and Lake Forest Park. He enjoys exploring the city by bus and foot.